At Bahia Sul Celulose’s paper mill facility in Mucuri, Brazil, large demineralized water usage required the mill to look for cost effective ways to minimize water production costs. In addition to cutting costs, the mill sought to improve the reliability of the demineralized water supply and increase water production capacity.
Located in the southern part of the Bahia state in Brazil, the Bahia Sul facility produces 570,000 tpy of cellulose. The source of make-up water for the mill is the Mucuri River, which offers treatment challenges due to high levels of microscopic organisms and total dissolved solids (TDS) throughout the year. Because it was very difficult to make the water acceptably clean using traditional filtration and chemical dosing, the mill installed a reverse osmosis (RO) water purification system in 1991 to eliminate biological contaminants.

Figure 1Pre-treatment of water prior to use in the reverse osmosis system at Bahia Sul.
However, the mill eventually found that its RO system was unreliable and the cleaning frequency was very high because of high levels of biofouling. By the end of 1998, the condition of the installed membranes, some of which were designed for brackish water, was such that a replacement was required.
After discussions with one of its membrane suppliers, Bahia Sul decided to try state-of-the-art fouling resistance (FR) membrane technology in one of its RO system trains, as well as new, standard membranes in another train. Taking a total system approach for improving RO system reliability, the mill combined this technology with special operating and cleaning conditions. Since March 1999, the two upgraded trains have performed consistently and at long intervals to reduce chemical use while increasing production of quality water.
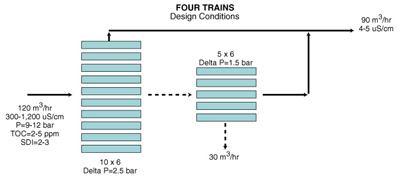
Figure 2: Reverse osmosis system configuration at Bahia Sul.
BIOFILM IMPACT ON INDUSTRIAL WATER. The development of biofilms and the role they play in membrane processes may be the most misunderstood and underestimated factor in the treatment of surface water and other industrial water systems by reverse osmosis/NanoFiltration technology. Simply stated, a biofilm consists of microbial cells (algal, fungal, or bacterial) and the extracellular biopolymer they produce.
Generally, bacterial biofilms are of most concern in RO industrial water systems, because they are responsible for the fouling of the membranes. This is due to the minimal amount of nutrients that are required for many species to proliferate at an alarming rate. Under ideal conditions, a single bacteria, reproducing every 20 minutes, can produce progeny in excess of 4.71 x 1021 within 24 hours.
Microorganisms can be found in both bulk water and on the surfaces of industrial water systems. Bacteria attach to surfaces by proteinaceous appendages, and once they have “glued” the cell to the surface, detachment of the organism is very difficult. One reason bacteria prefer to attach to surfaces is that the organic molecules adsorbed there provide nutrients. Once “stuck”, the organisms begin to produce material termed extracellular biopolymer or “slime”. The amount of biopolymer produced can exceed the mass of the bacterial cell by many times. The extracellular polymer produced may tend to provide a more suitable protective environment for the survival of the organism.
Once bacteria begin to colonize surfaces and produce biofilms, numerous symptoms begin to appear in the RO system, including:
• Gradual increase in feed pressure to the RO system
• Gradual increase in first stage delta P (in the two-stage RO system, delta P is the pressure of the first stage of the RO membrane)
• Reduced normalized permeate flow
• Performance improvement of the unit after high pH cleaning
• Characteristic odor of the membranes
FOULING RESISTANCE MEMBRANE TECHNOLOGY. Make-up water at Bahia Sul is pre-treated by taking water from the Mucuri River and passing it through a chlorine detention tank to prevent algae and bacteria growth and by oxidizing and breaking up organic compounds found in the water. The chlorinated water is then mixed with ferric sulfate and lime prior to entering the precipitator. The precipitator clarifies the water, which is then filtered through gravity sand filters. The clarified, filtered water is next passed through activated carbon filters that remove organic matter and traces of chlorine, which may remain in the treated water (Figure 1).
After pre-treatment, water is transferred to the mill’s RO system to eliminate biological contaminants and to purify the water down to the molecular level. The four RO trains at Bahia Sul were installed in 1991. Figure 2 shows the system configuration. The RO system is rated at 80 m3/hr per train in order to supply two mixed bed demineralizer trains. Currently, Bahia Sul produces a total of 555 tons/hr of steam or 280 m3/hr of demineralized water.
In the past, the RO system utilized Dow Chemical’s Filmtec BW30-400 and BW30-330 standard elements for brackish water, as well as other membrane types. By the end of 1998, the conditions of the installed membranes were such that a replacement was required. The plant was unreliable and the cleaning frequency was very high because of high levels of biofouling that built up a high pressure drop on the system.
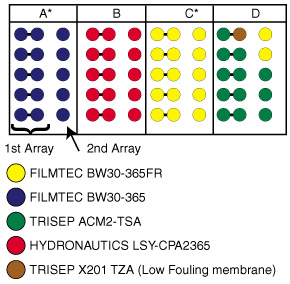
Figure 3Type of membranes employed at Bahia Sul, including fouling resistance membranes in Train C.
After discussions with its supplier, Bahia Sul decided to try Filmtec state-of-the-art fouling resistance (FR) technology in one of the trains. By March 1999, new membranes were installed in two trains at Bahia Sul. Train C, which had a capacity of 65 m3/hr, had its older Filmtec elements replaced by BW30-365 FR2 fouling resistance elements. However, for comparison purposes, standard BW30-365 elements were installed on Train A, which also had a capacity of 65 m3/hr. Figure 3 shows the types of membrane elements employed at Bahia Sul during 1999, while Figure 4 shows the product specifications and basic design of the new fouling resistance membranes in Train C.
In conjunction with the new membrane technology, special operating and cleaning conditions were instigated to form a total system approach. The reasons for choosing this approach were to increase tolerance to high organic matter and to improve water quality. Other reasons were to increase plant reliability and to increase the lifetime for membranes, thereby reducing cost.
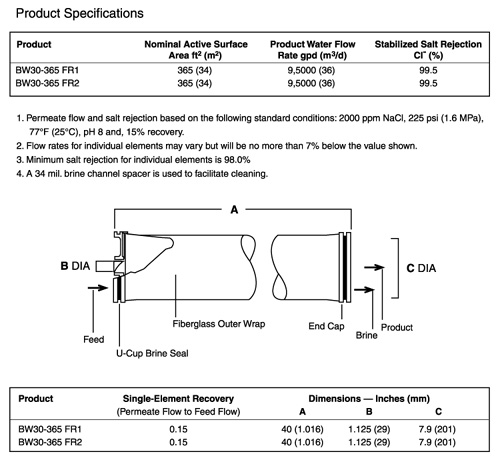
Figure 4Design and specifications of the new fouling resistance membranes used in Train C of the reverse osmosis system.
OPERATING AND CLEANING CONDITIONS. As most surface waters, the Mucuri River suffers a huge change in terms of organic matter (OM) levels and total dissolved solids (TDS) throughout the year. For example, OM could change between 1 ppm to 10 ppm and TDS between 250 ppm to 700 ppm. It is very difficult to absorb these changes in the pretreatment, and that is the reason why a fouling resistance membrane is desirable. However, it is clear that any membrane technology could not operate under these conditions for an extended period of time without significant performance decline.
In order to have a close follow up on the system, a special program was established by the mill with Dow Chemical to define specific operating conditions and cleaning procedures. The following operating conditions were established:
• Reject flow should be higher than 20 m3/h in order to achieve a turbulent flow on the brine channel to decrease the possibility of fouling (sweeping effect). This factor is related to the flux through the membrane surface.
• Maximum permeate flow should be 65 m3/h to 80 m3/h.
• Maximum % recovery should be at 78% (permeate flow / feed flow x 100)
• Clean the membranes when pressure drop increases by 15%.
• Clean array per array. At the beginning, it was not possible to perform the cleaning array per array; however, Bahia Sul modified the pipelines in order to allow this method.
• Maximum pressure drop by stage = 4.2 bar (avoiding membrane telescoping)
• Maximum flux at 13 gfd (gallons per square feet per day = 22.23 l/m2/h)
• After reviewing feed water composition and RO recovery, anti-scalant dosage was decreased from 3.75 ppm to a range 0.65 ppm to 1.0 ppm.
• Collect data required for normalization purposes. All the data is normalized in order to see if the RO unit performs unexpectedly.
A special computer program, provided by the supplier, has been used to normalize the data. Besides the above information, total oxidizable carbon (TOC) is being measured once a week and the silt density index (SDI) is measured daily.
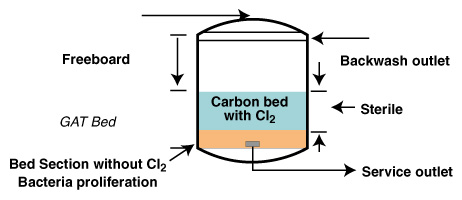
Figure 5Elevated bacteria levels are problematic in carbon beds without an appropriate sanitation program, resulting in biofouling of the RO membranes.
ADVANCED CLEANING PROCEDURES. Because of the low feed flow through the carbon filters and the lack of frequent sanitation, a microbiologic study was done in May 1999 to review the performance of the carbon filter. Levels of bacteria (cfu/ml) were measured before and after the carbon beds.
Bacteria levels of 0 cfu/ml were found before the filters and between 1 x 103 cfu/ml and 1 x 104 cfu/ml after the carbon beds. This is a common problem in carbon filters without an appropriate sanitation program, as at Bahia Sul (Figure 5). The carbon bed will remove any residual chlorine in the top portion of the bed. The bottom portion of the carbon bed will contain a significant amount of organic species and will promote rapid bacteria growth. This ready source of bacteria will eventually result in bio-fouling of the RO membranes.
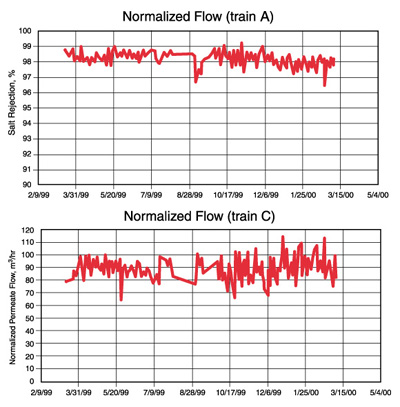
Figure 6 Normalized flow for Train A (standard membrane) and Train C (fouling resistance membrane).
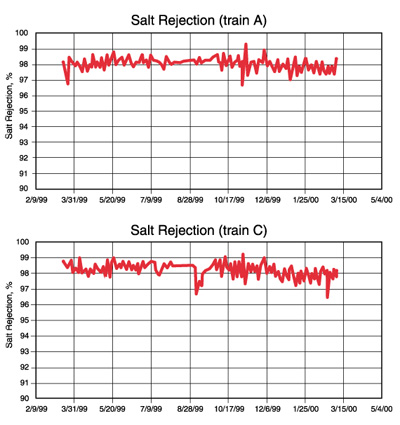
Figure 7Salt rejection for Train A (standard membrane) and Train C (fouling resistance membrane).
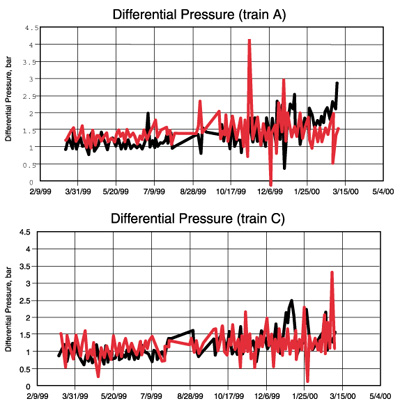
Figure 8Differential pressure for Train A (standard membrane) and Train C (fouling resistance membrane).
Because of the high biofouling potential, the following cleaning methodology has been employed at Bahia Sul:
1. Clean membranes array per array.
2. Flush membranes with permeate water.
3. Use high pH cleaning (NaOH, DDS, pH 12, 25° C to 30° C, employ color analysis of the cleaning solution).
4. Flush membranes again with permeate water.
5. Use low pH cleaning (HCl with pH 2 to pH 3).
6. Flush again with permeate water.
7. Use DBNPA disinfection** (2,2-dibromo-3-nitrilopropionamide, pH 3 to pH 4,100 ppm to 200 ppm, 30 to 60 minutes)
8. Use high pH cleaning/DBNPA deactivation (NaOH, pH>9, 25° C to 30° C).
9. Flush again with permeate water.
** Once a week, DBNPA is injected during 30 minutes at 10 ppm to 100 ppm to control biofouling. The data on Number 7 are for cleaning procedures, not for biofouling control. The injection at 10 ppm to 100 ppm is done weekly, and the cleaning procedure could be done every two to three months, depending on performance. DBNPA is a non-oxidizing biocide providing broad-spectrum control of bacteria, fungi, yeast and true algae. DBNPA decomposes rapidly in aquatic environments and is environmentally safe. Microorganisms that come into contact with DBNPA are rapidly killed by a mechanism that appears to involve reaction with the protein fraction of the cell membrane and inactivation of enzyme systems. The majority of microorganisms are killed within 5 to 10 minutes.
Eventually, a criterion on when to stop the high pH cleaning was implemented. Using the standard platinum cobalt test (PTCO) a criterion on when to stop the high pH cleaning was implemented. This criterion was based on the color of the cleaning solution after passing through the membranes. After passing a first load, it was common to obtain a color between 300 to 800 hundredths at 440 nm. When color drops below 50, the high pH cleaning is stopped.
PERFORMANCE AND CONCLUSIONS. Trains A and C have shown excellent, consistent performance since startup in March 1999. The design throughput, pressure drop, normalized flow and water quality have been achieved with a product quality of < 6 micro siemens/cm on the permeate. Figure 6 through Figure 8 show salt rejection, differential pressure, and normalized flow performance from startup through 12 months of operation for Train A and Train C.
After one year operating, pressure drop in the first stage changed from 1.0 bar to 1. 8 bar in Train A (standard membrane) and from 1.0 bar to 1.5 bar in Train C (FR membrane). This represents a longer lifetime than expected for FR membranes, if it is taken into consideration that Train C has been working to produce 20% more permeate water than Train A, as shown in Figure 6. Salt rejection and permeate flow are almost the same for both membranes.
After reviewing feed water composition, RO recovery (recovery = permeate flow/feed flow x 100), and Langelier Index (to predict scaling potential for CaCO3), the mill decided to reduce consumption of anti-scaling chemicals by 9,000 liter/year, which amounts to a savings of $36,000/year.
In addition, since the RO system has been functioning with the new membranes and operating conditions, the regeneration frequency of the demineralizers has been decreased substantially. The mill now regenerates the demineralizers about every 12 days, compared with six days prior to the installation of the new membranes and operating conditions. By extending the time interval between regeneration, Bahia Sul expects to eliminate the use of 14,250 liters of hydrochloric acid (35%w = 16.7 tons ) and 17,100 liters (26 tons) of sodium hydroxide 50 %, annually. As a result of the excellent performance obtained, Bahia Sul decided to replace one more train with the second generation fouling resistance membranes this year.
Bahia Sul attributes the success of this project to the total system approach that was taken to improve RO system reliability. This approach included the following factors: special operating conditions, new cleaning and disinfection procedures, state-of-the-art fouling resistance membrane technology, close monitoring/analysis of system performance, and commitment and training of those involved in the project.
BIBLIOGRAPHY
1. FILMTEC Membranes, Technical Manual. The Dow Chemical Company.
2. Zahid Amjad, Reverse Osmosis; Membrane Technology, water Chemistry and Industrial Applications. Van Nostrand Reinhold, New York 1992.
3. Coker, Steven. Winning The Battle Against Biofilm Formation. The Dow Chemical Co, Technical literature Form No. 609-00261-199x
4. The Dow Chemical Co, FT30 Reverse Osmosis Membrane Biological Protection and Disinfection, Technical literature, Form No. 609-24010-498QRP
Sinésio Carvalho Soalheiro is water treatment supervisor for Bahia Sul Celulose, Mucuri, Brazil.

|